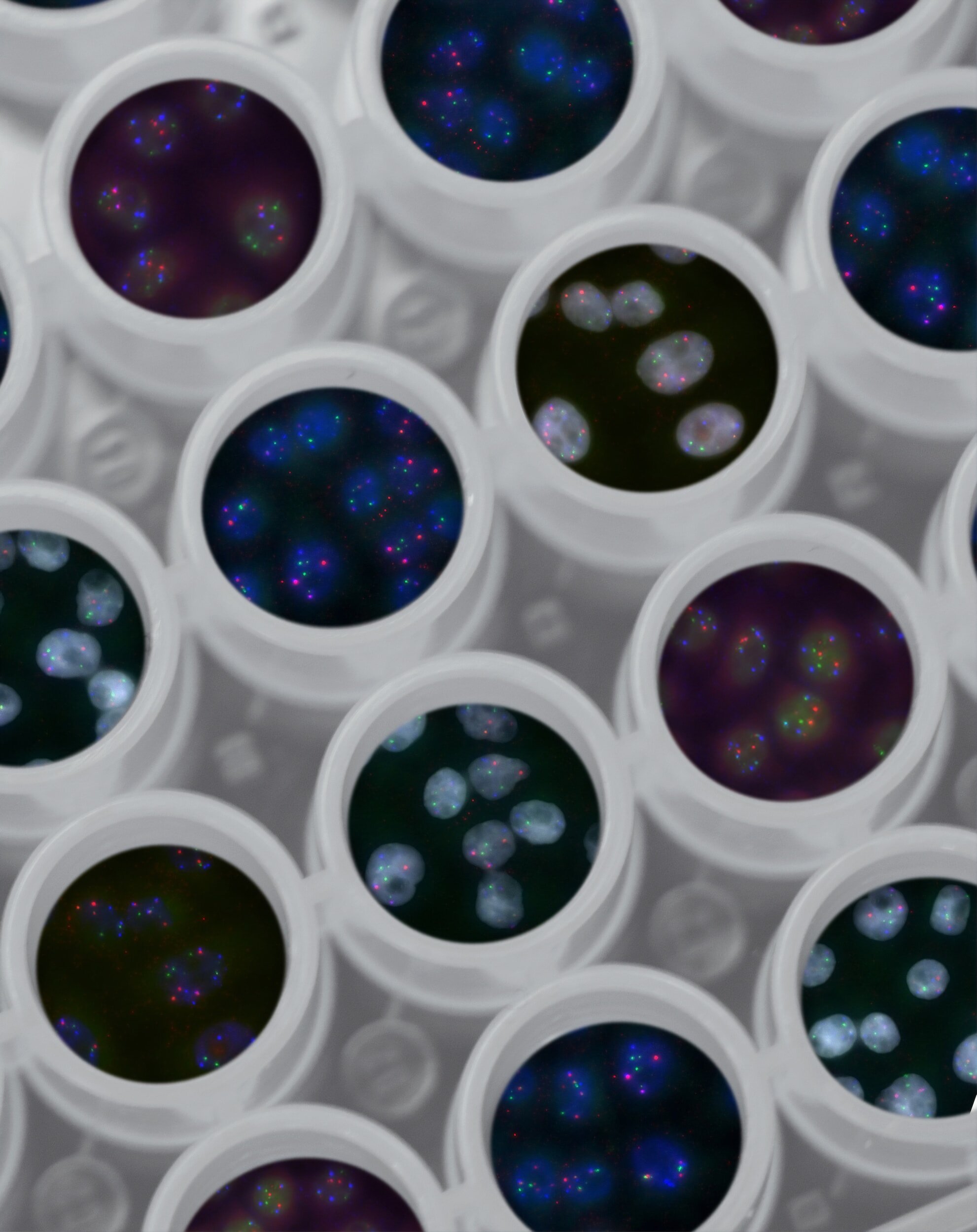
RESEARCH
“Sapere Aude”
Spatial Genome Organization in Health and Disease
The overarching goal of our research group is to understand how the positioning and movement of DNA inside the cell’s nucleus modulates genome expression and stability. We also explore connections between these fundamental cellular processes and human diseases, especially in the context of cancer, aging, and related diseases. Our research combines yeast genetic models with different mammalian cell systems and mouse models.
Research Cluster 1 - Nuclear compartmentalization in health, cancer, and aging
We have been exploring broader roles for nuclear compartmentalization and related non-coding RNAs (ncRNAs) in the modulation of genome expression and stability. We highlight here some of our key findings. First, we uncovered different protein networks maintaining yeast cellular lifespan by tethering distinct types of repetitive DNA loci to the nuclear envelope [Chan, J.N. et al. Dev. Cell (2011)]. Next, we found that the RNA-binding protein complex Pbp1-Pbp4-Lsm12 binds to long intergenic ncRNAs (lincRNAs) emerging from spacers near yeast rRNA genes to prevent these lincRNAs from post-transcriptionally forming genome-destabilizing and lifespan-shortening triplex nucleic acid structures called R-loops [Salvi, J. et al. Dev. Cell (2014)]. Importantly, in this and another study, we uncovered a mechanism through which caloric restriction hyperactivates the R-loop suppressors RNase H1, RNase H2, and Pif1 to counter excessive R-loop accumulation and restore lifespan in cells lacking other R-loop suppressors, including yeast Pbp1 and human BRCA2 [Salvi, J. et al. Dev. Cell (2014); Abraham, K.J. et al. Nucleic Acids Res. (2016)]. Intriguingly, S. cerevisiae Pbp1 is the orthologue of human ATXN2, a protein with a polyglutamine (polyQ) tract. The moderate and excessive expansion of this polyQ tract increases the risk of developing amyotrophic lateral sclerosis (ALS) and is the genetic cause of spinocerebellar ataxia type 2 (SCA2), respectively. ALS and SCA2 are severely debilitating and often lethal neurodegenerative disorders, but how polyQ expansion alters the cell remains unclear. To speed up research in this area, we created powerful yeast genetic models of ATXN2 polyQ expansion [Ostrowski, L.A., et al. Commun. Biol. (2018)]. Surprisingly, yeast cells with SCA2-modeling polyQ expansions in Pbp1 exhibited a shorter lifespan than that of Pbp1 knockout cells, pointing to a gain of toxic function. Indeed, we have shown that SCA2-modeling Pbp1 proteins form liquid and solid aggregates that localize to the nucleolus, where they sequester chromosome-stabilizing and lifespan-maintaining factors. In addition, we reproduced findings from our SCA2-modeling yeast in human cell models of SCA2, including patient-derived cells. We also discovered human nucleolar RNA Pol II and how it battles intergenic RNA Pol I to prevent excessive levels of sense intergenic ncRNAs (sincRNAs) accumulation that otherwise dismantle nucleoli and abrogate their role in ribosome biogenesis [Abraham, K.J. et al. Nature (2020); Khosraviani, N. et al. BioRxiv (2023)]. On another front, we have applied our expertise in genome expression and stability to the study of the COVID-19-causing SARS-CoV-2. By collaborating with experts in virology and proteomics, we revealed that SARS-CoV-2 targets the process of ribosome biogenesis in human cells, informing existing and future therapeutic development efforts focused on this and related pathogens [Yerlici et al. Cell Rep. (2024)].
Research Cluster 2 - Nuclear and genome dynamics impacting DNA repair in cancer, aging, and related diseases
In this research theme, we adopt a multidisciplinary approach that brings us together with different engineers working at the forefront of aerospace, mechanical, and industrial engineering. This collaborative effort aims to decipher the motions of damaged DNA inside the yeast or human nucleus and to understand how such motions are controlled, and how they impact genome stability and cell survival.
This effort was initiated in my laboratory a few years ago, when damaged was shown to exhibit increased random mobility inside the cell nucleus, somehow promoting DNA repair. This motion is now thought to promote DNA repair by allowing damaged DNA loci to find homologous DNA sequences for repair, escape repair-repressive heterochromatin domains, and/or to move to repair-conducive nuclear domains such as the nuclear pore complexes. This increased motion of damaged DNA has been observed in yeast, fly, worm, mouse, and human cells to various levels. Regardless of the organism, the motion of damaged DNA appeared to be random, or lacking direction. To better understand what dictates the motion of damaged DNA, we first conducted a targeted yeast genetic screen, which identified the Kinesin-14 motor protein complex as being highly important for the mobility of damaged DNA. Indeed, we reported that signaling via the DNA damage response remodels chromatin and allows many damaged DNA sites to recruit Kinesin-14, which is required for the mobilization of the damaged sites to the nuclear pore complexes for repair [Chung, D.K. et al. Nature Commun. (2015)]. We dubbed Kinesin-14 the first molecular DNA ambulance, a term that is growing in popularity in the literature. This finding had two immediate implications. It suggested that motor protein complexes mobilizing damaged DNA may be doing so by moving onto molecular autobahns such as actin or microtubule filaments. Indeed, we reported that the yeast Kinesin-14 motor protein complex mobilizes damaged DNA onto nuclear microtubule filaments laid on demand upon the induction of DNA breaks [Oshidari, R. et al. Nature Commun. (2018)]. We call these filaments DNA damage-inducible intranuclear microtubules (DIMs). Moreover, in this study, we collaborated with scientists at the University of Toronto Institute for Aerospace Studies to closely examine if the motion of damaged DNA is truly random. Importantly, we revealed that damaged DNA exhibits complex yet directional motions, which appear to spiral and oscillate in a manner that reflects motor protein function and the movement of DIMs inside the nucleus. This reconciled the apparent disagreement between the role of motor proteins in damaged DNA mobility and the previous, yet inaccurate, assumption that damaged DNA loci move in manner that is always random. More recently, we reported that a broader network, which is composed of various nuclear filaments and DNA repair protein liquid droplets, mediates the clustering of damaged DNA sites to create the so-called DNA repair center [Oshidari, R. et al. Nature Commun. (2020)]. Our recent work has also uncovered the process of DNA repair by nuclear metamorphosis, or nucleomorphosis [Shokrollahi, M., Stanic, M., Hundal, A. et al. Nature Struct. Mol. Biol. (2024)]. In this process, a complex nuclear-cytoplasmic DNA damage response creates DSB-capturing nuclear envelope tubules (dsbNETs), which bring the envelope with its repair-conducive capacities to damaged DNA throughout the nucleus to promote repair. We are currently exploiting this mechanism to develop novel anti-cancer and anti-aging therapeutics.
HIGHLIGHTED DISCOVERIES
DNA repair by nucleomorphosis and dsbNETs (Nature Struct. Mol. Biol. 2024)
Nucleolar RNA Pol II and how it regulates human ribosome biogenesis (Nature 2020; BioRxiv 2023)
DNA repair by a network made of various nuclear filaments and Rad52 repair protein liquid droplets (Nature Commun. 2020)
DNA damage-inducible intranuclear microtubule filaments, DIMs (Nature Commun. 2018)
Protein trans-aggregation and the first yeast genetic models of ALS and SCA2 (Commun. Biol. 2018)
First molecular DNA ambulance, Kinesin-14 (Nature Commun. 2015)
The direct role of the nuclear envelope in genome stabilization (Nature 2008)
Lifespan maintenance via perinuclear tethering of rDNA and telomeric repeats (Dev. Cell 2011, Aging Cell 2012)
Conserved role of Ataxin-2 in R-loop control (Dev. Cell 2014, Nucleic Acids Res. 2016)
SARS-CoV-2 targets ribosomal RNA production (Cell Rep. 2024)